Klaus Gramann
Research Interests - Mobile Brain/Body Imaging (MoBI)
MoBI in the news:
Discover Magazine
UC Newsroom
CalIT2 Newsroom
CalIT2 Newsroom
HMC Architects
Health Care IT News 2008
Health Care IT News 2009
Health Care Design
MoBI Publications:
Gwin,
J.T.,
Gramann,
K., Makeig, S., & Ferris,
D.P. (1011). Electrocortical
activity
is coupled to gait cycle
phase during treadmill walking.
NeuroImage, 54, 1289-1296.
Gwin,
J.T.,
Gramann,
K., Makeig, S., & Ferris,
D.P. (2010). Removal
of
movement
artifact
from
high-density
EEG
recorded
during
walking
and
running.
Journal of
Neurophyiology, 103,
3526-3534.
Gramann, K., Gwin, J.T.,
Bigdely-Shamlo, N.,
Ferris, D.P., & Makeig, S.
(2010). Visual
evoked
responses
during
standing
and
walking.
Frontiers in Human Neuroscience, 4:202.
Makeig, Gramann,
Jung, Sejnowski,
&
Poizner (2009). Linking
Brain,
Mind, and Behavior.
International
Journal of Psychophysiology, 73(2), 95-100.
Gwin, J.T., Ferris, Makeig, S., & Gramann, K. (2010). Imaging the
mobile brain. Poster at the
Society for Neuroscience Conference 2010.
____________________________________________________________________________________________________________________________________________________________________________________________
MoBI - A
New Method to Investigate Active Human Cognition
My
research on high definition mobile EEG-recordings attempts
to overcome the traditional restrictions of brain imaging by applying
new developments in EEG-recording techniques and analyzes approaches
(Makeig et
al., 2002; Delorme & Makeig, 2004) to Natural Cognition, analyzing
ambulatory
recordings
of brain electrical activity
during actions in natural space with complex experimental designs.
Natural Embodied Cognition,
including self-determined information
uptake and motor action to orient in space is recorded in small
and large scale environments. Recordings of active behavior will
inevitably
be
confronted with eye- and muscle activity considered artifactual. Our
analyzes have to deal with complex motor behavior not encountered
hitherto due
to the restrictions of classical brain imaging analyzes. More
specifically, subjects move in space including whole body
movements as well as movements of the upper limbs and the head to
reach a goal or actively search for relevant information needed to
guide behavior. This kind of behavior includes the coordinated
activation of sets of muscles (i.e., muscles of the neck) directly
assessed by high-density EEG-recordings, introducing functional
non-brain activity associated with movement and accompanying
cognitive processes. See
video recording of an experimental session using the
high-definition Mobile Brain/Body Imaging (MoBI)
technique.
The first and most important question is whether the new MoBI
allows recording of brain dynamics during active motor
behavior. The answer is - YES.
To prove this point we conducted a feasebility study (Visual
evoked
responses
during
standing
and
walking) and had participants stand, walk, and
run on a treadmill while attending to a visual oddball paradigm. In
this study we demonstrated that we can replicate the visual P300
associated with processing of target-stimuli while subjects acitvely
move on a treadmill. ICA was able to decompose brain and non-brain
activity without advanced a priori artifact rejection methods while
subjects walked with speeds up to 1.3 m/s. Even when subjects were
running on a treadmill, we were able to use ICA to analyze the brain
dynamics after the raw data was artifact rejected using approaches
similar to the rejection of fMRI artifacts in parallel EEG-fMRI
recordings (see Removal
of
movement
artifact
from
high-density
EEG
recorded
during
walking
and
running). Here is a link to a
video showing a participant
running on a treadmill at Dan Ferris' Human Neuromechanic
Laboratory, University of Michigan. The general setup and the results
from ICA are explained below.
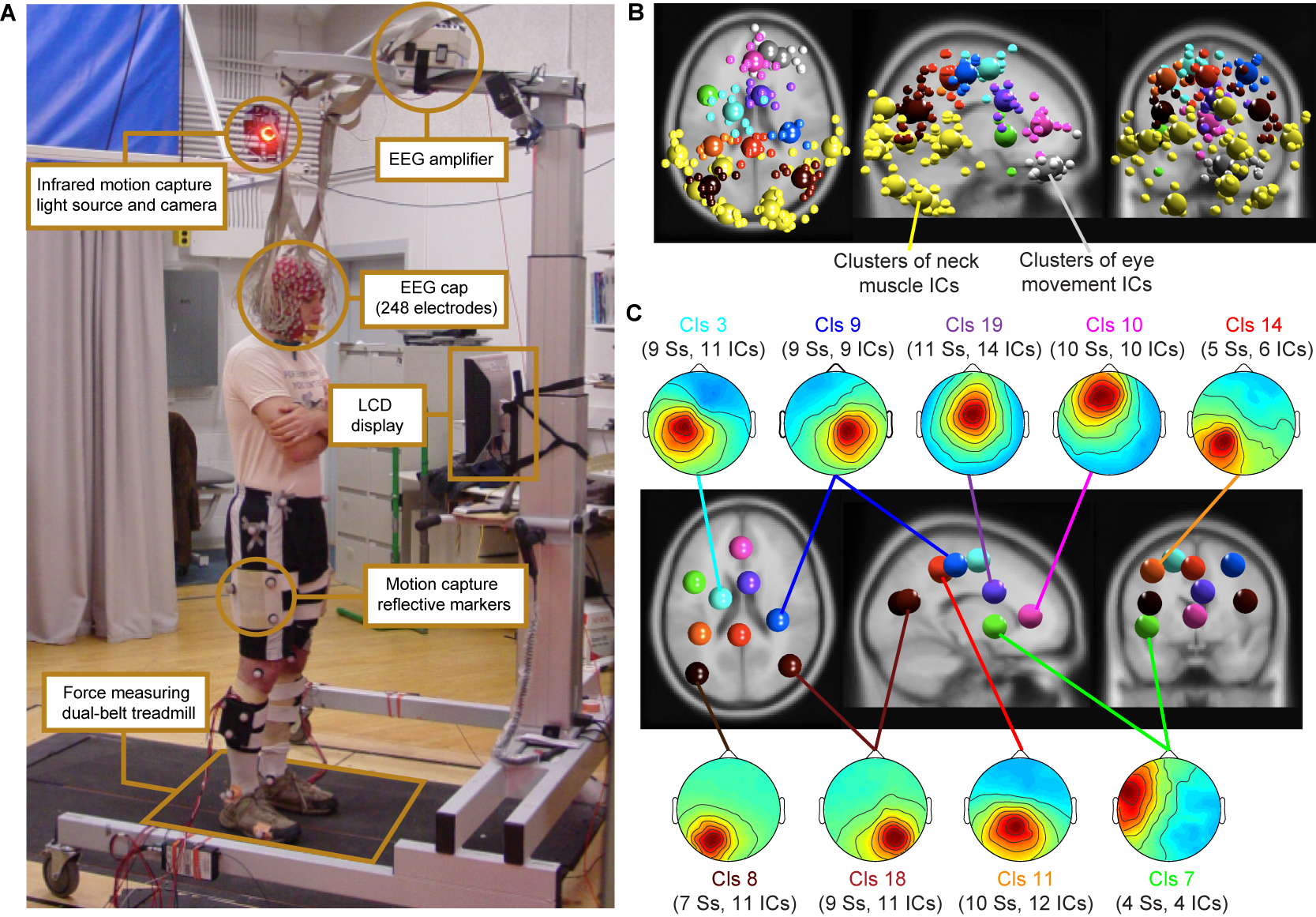
Figure 1: (A)
Experimental setup: subject standing on the dual-belt treadmill facing
the LCD display. Components of the experimental setup are highlighted
and described in the linked text boxes. (B) Equivalent-dipole locations of
independent component (IC) processes (small spheres) and IC cluster
centroids (large spheres) projected on horizontal, sagittal, and
coronal views of the standard
MNI brain. (Yellow) Neck-muscle ICs; (gray) eye-movement
ICs;
(other colors) brain-based ICs (C)
(Scalp maps) Mean projections to the
scalp of the indicated brain-based IC clusters. Labels give
the index (Cls #), number of subjects
(Ss), and number of independent components
(#
ICs) for each cluster.
Comparing the P300 for target and non-target trials for participants
standing, slow walking, and fast walking we demonstrated that it is
feasible to record and analyze brain dynamics while subjects avctively
move.
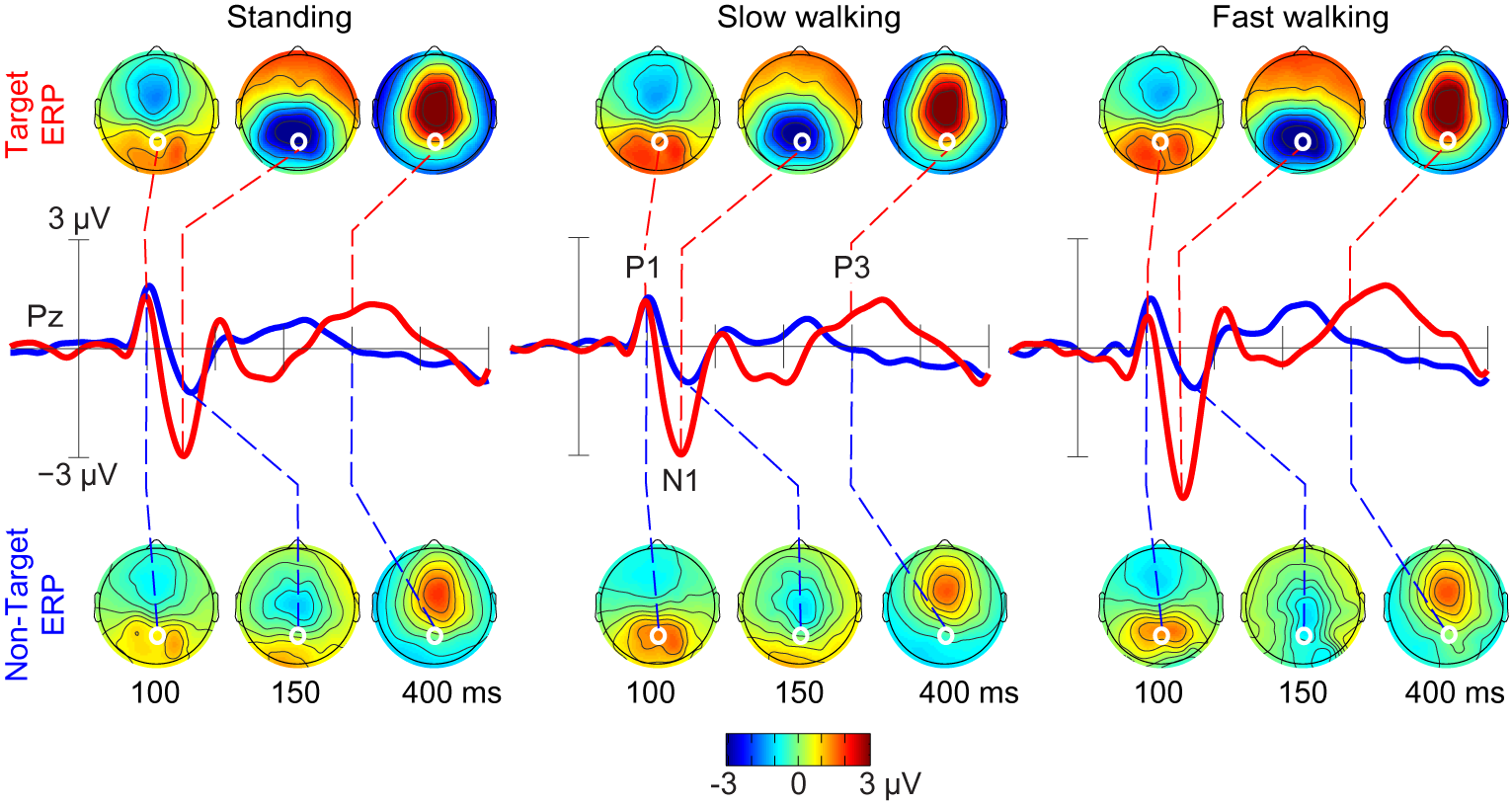
Figure 2: Grand-average ERPs following ICA-based artifact removal in
the standing, slow walking, and fast walking conditions. Middle-row
traces show ERP time courses at electrode Pz (red, target ERPs; blue,
non-target ERPs). Scalp maps show the grand-average ERP scalp
distributions at 100, 150, and 400 ms after onsets of target stimuli
(upper row) and non-target stimuli (lower row). White dots indicate the
location of electrode Pz. Note the scalp map similarities across
movement conditions.
With this first study we have demonstrated the feasibility of MoBI studies
of
event-related EEG dynamics in subjects performing
full-body movements in a 3-D environment.
Future MoBI studies will address
critical questions concerning macroscopic brain dynamic
patterns
supporting motivated motor behavior and
more general aspects of embodied
cognition. Answers to many questions
that
were formerly not possible to investigate using brain imaging may
now be approached. For example: How are eye movements, head movements
and brain activity accompanying attentional orienting interrelated?
What are the accompanying brain
activity of spatial cognitive processes while subjects experience
natural vestibular and proprioceptive feedback associated with heading
changes during navigation? New information available from detailed
analysis of concurrently recorded EEG and body motion data, an imaging
modality we refer to as MoBI, should
open
new avenues for analyzing the association of brain dynamics with
specific aspects of movement and motivated action.
New Insights into Human
Cognitive Neuroscience
The results of a second experiment demonstrate the potential of this
new
imaging method. In this experiment, subjects were required to
point to, look to, or walk and subsequently point to one out of 6
possible objects lovcated around the subject in a semicircular arrey
(see Figure 1).
Figure 3: Experimental setup of the first MoBI experiment.
Subjects were standing in a large room facing a computer screen at a
distance of approxemately 3.5 meters. Six obejcts were located in a
semicircular array around the subject. Data from EEG and motion capture
were recored und synchronized online via the DataRiver Software
(Vankov, 2009). When the subject pointed to the monitor or one of the
other objects, the vector going through the motion capture
sensors placed on the pointing finger and the forehead of the subject
was registered online relative to the 3-D coordinates of the object
locations. If the vector entered a hot spot defined around the objects,
a feedback was given and the trial terminated. With the next pointing
movement towards the central monitor the next trial was initiated. Each
trial was composed of several subsequent displayes, starting with a
fixation cross for 3 seconds, followed by the display of one of the six
objects for 1 second, with a subsequent fixation cross of 3
seconds, followed by tyhe indication of three possible actions to
perform (look to, point to, or walk to), which was followed by a third
fixations cross for 3 seconds. When this last fixation cross changed
its color from black to red, the subject was allowed to react. The
other half of the trials were identical with the action instruction
being displayed first and the object being displayed second.
To demonstrate that we
record the same brain dynamics during
passive cognition we have to replicate earlier findings that were
recorded using traditional EEG-approaches. One example of a well
known EEG-phenomena is the motor-mu, or alpha-desynchronization over
motor cortex when subject prepare or imagine movements of the limb (described
by
Pfurtscheller
and
Aranbiar, 1979). Analyzing the brain dynamics of
subjects during passive
cognition (while the subject is standing, looking at the central
display perceiving the instructions on the monitor) we can compare our
data to the data from traditional recordings. The results can be seen
in figure 2 below.
Figure 4:
Upper row displays a cluster of independent component processes
(small red balls) with the centroid of the cluster (big red ball)
located in or near the motor cortex (data from 8 subjects). The cluster
is shown from a horizontal, sagittal, and coronal view. The second row
displays the trial sequence as described above, starting with a
fixation cross. The last row displays mean cluster event-related
spectral perturbation in dB in log-frequency scale from 3 to 150 Hz,
revealing significant deviation from baseline (warm colors indicate
power increase, cold colors indicate power decrease) over the entire
time period of nine seconds and a short period of time thereafter.
As can be seen in Figure 2, the event-related spectral pertubation
pattern in motor cortex replicates the well known motor-mu
desynchronization. While the power decrease is absent during the first
fixation cross, power in the 10 Hz and first harminc frequency bands
starts to decrease (copmpared to baseline as derived from the first
second of the fixation cross) with onset of the first relevant
information screen. During the time period of the second fixation
cross, motor-mu desynchronization seems to oscillate and then decreases
further with onset of the second display. After this - which is the
point in time when subjects know where to and what to do - the
desynchronization reaches it's maximum. This pattern of brain dynamics
in motor cortex can be seen as replication of the traditional
experimental setting with a desynchronization of motor-mu activity
while subjects prepare voluntary movements.
Of course that still does not proof whether we can record and analyze
the brain dybnamics during
active movements of the subject.
Figure 3 below displays the brain dynamics of the same motor cortex
cluster with onset of and during execution of movements from 8
subjects. Subjects were looking or pointing (including natural looking)
to the 6 different
objects
(the
data
shown is averaged across all objects) which included
rotations of the head and upper body to the left and right up to 80
degrees.
Figure 5: Displays the identical independent component cluster
as dexcibed in figure 2 above seen from horizontal, sagittal, and
coronal slices. The small inset in the second row displays the tril
structure of one trial. The next inset shows the last 500 msec of the
last fixation cross and the time period with onset of the red fixation
cross (time point 0 in the figure above) indicating the imperative
stimulus. The last row displays event-related spectral pertubation (in
dB) in log-frequency from 3 to 150 Hz.
The
results reveal that several brain areas demonstrate remarkable
sensitivity in their dynamics accompanying different phases of a
movement. In other words, timing of motor behavior is reflected in
brain dynamics.
____________________________________________________________________________________________________________________________________________________________________________________________